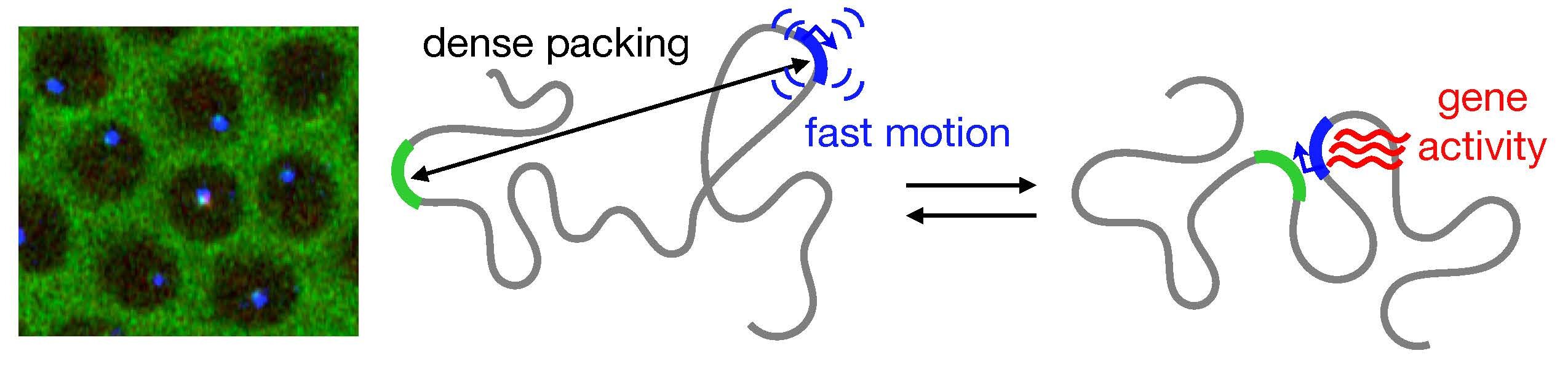
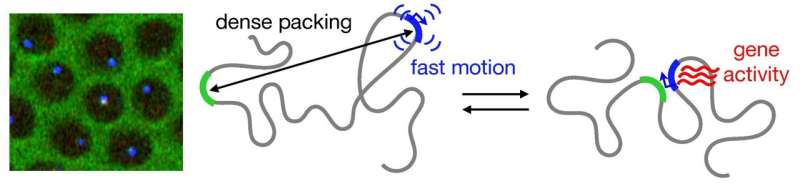
Despite being densely packed to fit into the nucleus, chromosomes storing our genetic information are always in motion. This allows specific regions to come into contact and thereby activate a gene. A group of scientists from the Institute of Science and Technology Austria (ISTA), Princeton University, and the Institut Pasteur in Paris has now visualized this dynamic process and gives novel insights into the physical characteristics of DNA.
Performing cutting-edge science requires thinking outside the box and bringing together different scientific disciplines. Sometimes this even means being in the right place at the right time. For David Brückner, postdoctoral researcher and NOMIS fellow at ISTA, all the above-mentioned things came into effect as he attended an on-campus lecture by Professor Thomas Gregor from Princeton University.
Inspired by the talk, Brückner reached out with an idea: to physically interpret the specific data sets Gregor presented. Now, the results of their collaboration are published in Science. They highlight the stochastic (random) motion of two specific gene elements on a chromosome, which have to come into contact for the gene to become active in 3D space.
How DNA fits into a cell nucleus
Living organisms like humans are built on genes that are stored in DNA, our molecular blueprint. DNA is a polymer, a huge molecule of smaller individual parts (monomers). It is located in every cell’s nucleus.
“Depending on the organism, the DNA polymer can be up to meters long, yet the size of the nucleus is on the order of microns,” Brückner explains. To fit into the tiny nucleus, DNA becomes compacted by being coiled as if on a spool, and further compressed into the well-known shape of chromosomes, which we have all encountered in a biology textbook.
“Despite being heavily condensed, chromosomes are not static; they are jiggling around all the time,” the physicist continues. These dynamics are very important. Whenever a specific gene must be activated, two regions on the polymer called “enhancer” and “promoter” must come into close contact and bind to each other. Only when this happens, cellular machinery reads off the gene’s information and forms the RNA molecule, which eventually gives rise to proteins that are essential for all the processes a living organism requires.
Depending on the organism, the enhancer and promoter can be quite far from each other on the chromosome. “With previously used methods, you could get a static view of the distance between these elements, but not how the system evolves over time,” Brückner explains. Intrigued by this missing information, the scientists set out to get a dynamic look at how these elements are organized and how they move in 3D space in real time.
Visualizing gene regions
To achieve this goal, the experimental scientists from Princeton established a method to track those two DNA elements over a certain time period in a fly embryo. Through genetic manipulation, the DNA elements were fluorescently labeled, with the enhancer region illuminating in green and the promoter in blue. Using live imaging (time-lapse microscopy of living cells) the scientists were able to visualize the fluorescent spots in fly embryos to see how they were moving around to find each other.
Once the two spots came into proximity, the gene was activated and an additional red light turned on as the RNA was also tagged with red fluorophores. Brückner excitedly adds, “We got a visual readout of when the enhancer and promoter got in contact. That gave us a lot of information about their trajectories.”
DNA is densely packed and exhibits fast motion
The challenge then was how to analyze this huge data set of stochastic motion. Brückner’s background in theoretical physics allowed him to extract statistics to understand the typical behavior of the system. He applied two different, simplified physical models to cut through the data.
One was the Rouse model. It assumes that every monomer of the polymer is an elastic spring. It predicts a loose structure and fast diffusion—a random movement, where occasionally the gene regions encounter each other. The other model is called the “fractal globule.” It predicts a very compact structure and therefore slow diffusion.
“Surprisingly, we found in the data that the system is described by a combination of these two models—a highly dense structure you would expect based on the fractal globule model, and diffusion which is described by the statistics from the Rouse model,” Brückner explains.
Due to the combination of dense packing and fast motion, the binding of these two gene regions depends much less on their distance along the chromosome than previously anticipated. “If such a system is in a fluid and dynamic state all the time, long-distance communication is much better than we might have thought,” Brückner adds.
This study brings together the worlds of biology and physics. For physicists, it is interesting, because the scientists tested the dynamics of a complex biological system with physical theories that have been around for a long time; and for biologists, it gives insights into the characteristics of a chromosome, which might help to understand gene interaction and gene activation in more detail.
More information:
David B. Brückner et al, Stochastic motion and transcriptional dynamics of pairs of distal DNA loci on a compacted chromosome, Science (2023). DOI: 10.1126/science.adf5568. www.science.org/doi/10.1126/science.adf5568
Provided by
Institute of Science and Technology Austria
Citation:
DNA organization in real-time: How the motion of DNA controls gene activity (2023, June 29)
retrieved 30 June 2023
from https://phys.org/news/2023-06-dna-real-time-motion-gene.html
This document is subject to copyright. Apart from any fair dealing for the purpose of private study or research, no
part may be reproduced without the written permission. The content is provided for information purposes only.