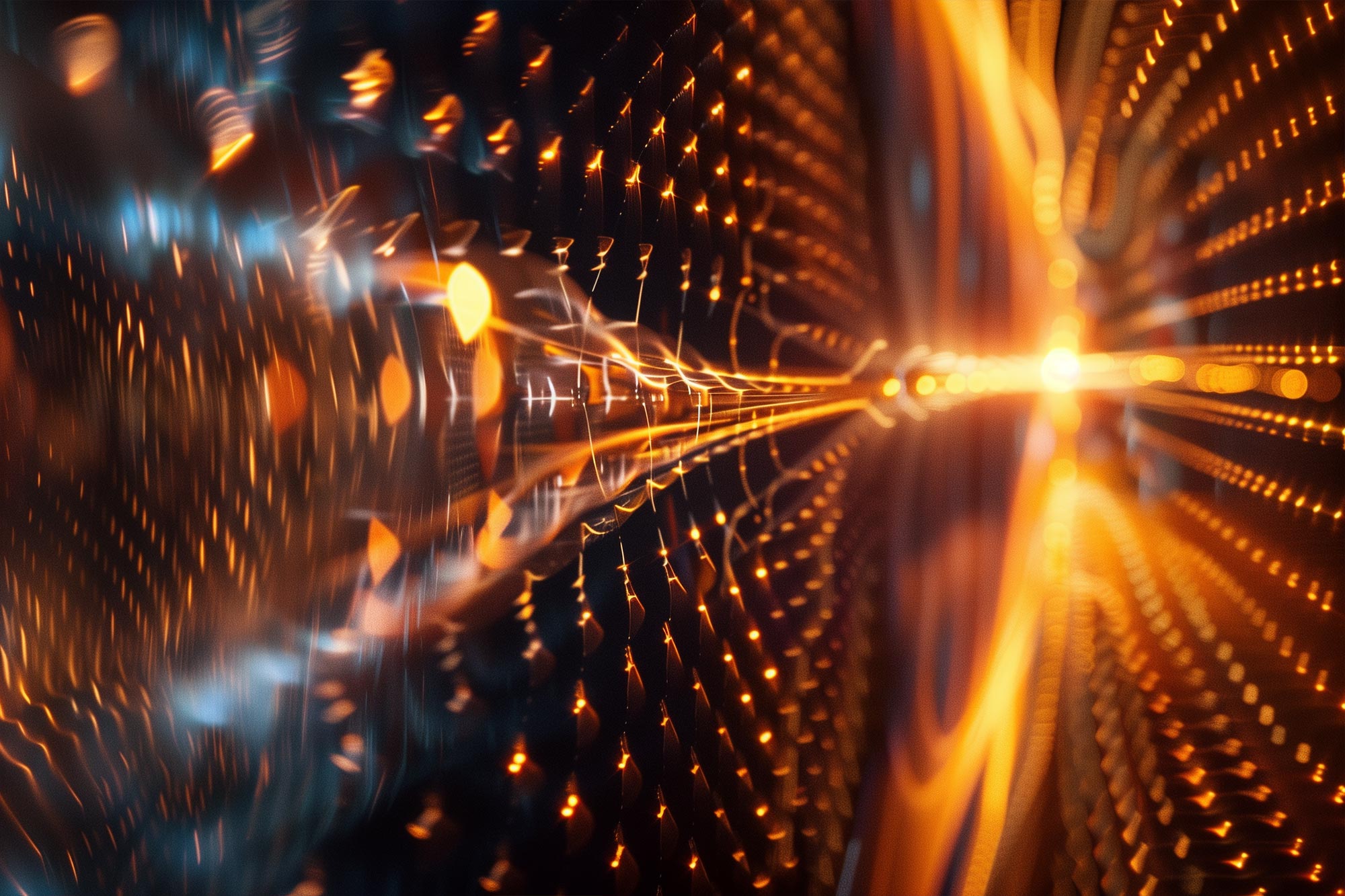
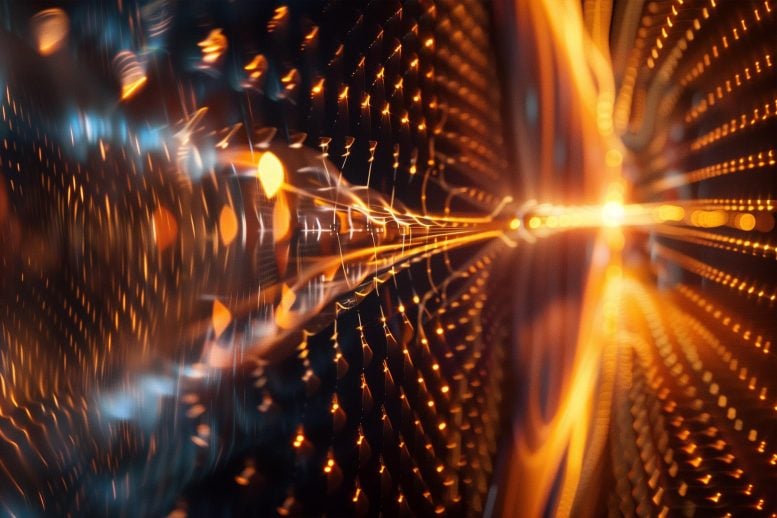
Ferroelectric material adapts to light stimuli by reorganizing atomic connectivity on the nanoscale in a way that resembles the plasticity of neural networks.
Researchers are exploring ferroelectric materials for their potential in energy-efficient microelectronics. These materials can adapt their structure in response to light pulses, creating a variety of domain structures that could revolutionize data processing and storage, aiming to significantly reduce the power consumption of supercomputers and data centers.
Discovering Adaptive Ferroelectric Materials
“Today’s supercomputers and data centers demand many megawatts of power,” said Haidan Wen, a physicist at the U.S. Department of Energy (DOE) Argonne National Laboratory. “One challenge is to find materials for more energy-efficient microelectronics. A promising candidate is a ferroelectric material that can be used for artificial neural networks as a component in energy-efficient microelectronics.”
Ferroelectric materials are commonly used in various information processing devices, such as computer memory, transistors, sensors, and actuators. Researchers at Argonne National Laboratory have discovered surprising adaptive behavior in a ferroelectric material, which can evolve step-by-step toward a specific outcome based on the number of photons from light pulses it receives. This research was conducted in collaboration with scientists from Rice University, Pennsylvania State University, and the DOE’s Lawrence Berkeley National Laboratory.
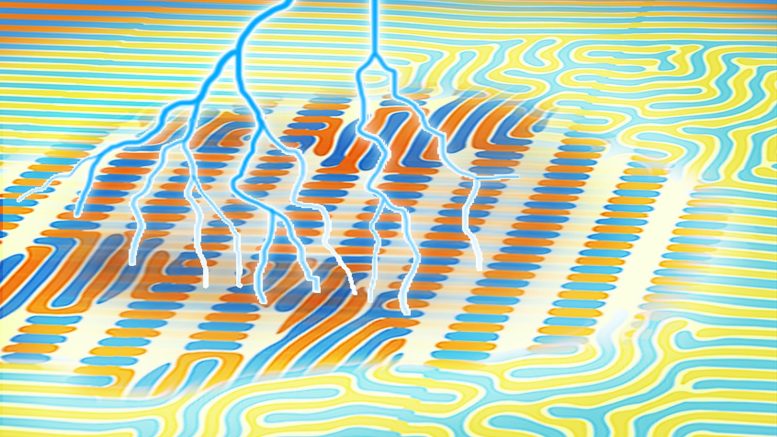
The material features networked islands, or domains, that are distinct from each other, much like oil in water. These domains, measured in nanometers (billionths of a meter), can rearrange themselves in response to light pulses. This adaptive behavior could be applied to enable energy-efficient data processing in microelectronics.
The team’s ferroelectric sample is structured as a sandwich of alternating layers of lead and strontium titanate. Prepared by the Rice University collaborators, this seven-layer sandwich is 1,000 times thinner than a piece of paper. Previously, the team had shined a single, intense light pulse on a sample and created uniform, nanoscale-ordered structures.
“Today’s supercomputers and data centers demand many megawatts of power. One challenge is to find materials for more energy-efficient microelectronics. A promising candidate is ferroelectric material that can be used for artificial neural networks as a component in energy-efficient microelectronics.”
Haidan Wen, Argonne physicist
Innovating With Light-Induced Nanodomains
“This time, we hit the sample with many weak light pulses, each of which lasts a quadrillionth of a second,” Wen said. “As a result, a family of domain structures, rather than a single structure, was created and imaged, depending on the optical dosage.”
To visualize the nanoscale responses, the team called upon the Nanoprobe (beamline 26-ID) operated by the Center for Nanoscale Materials and the Advanced Photon Source (APS). Both are DOE Office of Science user facilities at Argonne. With the Nanoprobe, an X-ray beam tens of nanometers in diameter scanned the sample as it was exposed to a barrage of ultrafast light pulses.
The resulting images revealed networked nanodomains being created, erased, and reconfigured due to the light pulses. The regions and boundaries of these domains evolved and rearranged at lengths of 10 nanometers — about 10,000 times smaller than a human hair — to 10 micrometers, roughly the size of a cloud droplet. The final product depended on the number of light pulses used to stimulate the sample.
Future Implications for Computing Efficiency
“By coupling an ultrafast laser to the Nanoprobe beamline, we can initiate and control changes to the networked nanodomains by means of light pulses without requiring much energy,” said Martin Holt, an X-ray and electron microscopy scientist and group leader.
The sample begins with a spiderweb-like arrangement of the nanodomains, and due to the disturbance created by the light pulses, the web breaks down and forms entirely new configurations that work in the service of some desired end in analogy to an adaptive network.
“We have discovered entirely new arrangements of these nanodomains,” said Stephan Hruszkewycz, an Argonne physicist and group leader. “The door is now wide open to many more discoveries. In the future, we will be able to test different regimes of light stimulation and observe even more unknown nanodomains and networks.” The power to visualize nanoscale change over time will be greatly improved with the recent upgrade to the APS, promising as much as 500 times brighter X-ray beams.
With this groundbreaking discovery of time-dependent changes in networked nanodomains, developers are on the path to building adaptive networks for information storage and processing. This advancement promises to create more energy-efficient computing systems.
This research is based on a paper in Advanced Materials.
Reference: “Optical Control of Adaptive Nanoscale Domain Networks” by Marc Zajac, Tao Zhou, Tiannan Yang, Sujit Das, Yue Cao, Burak Guzelturk, Vladimir Stoica, Mathew J. Cherukara, John W. Freeland, Venkatraman Gopalan, Ramamoorthy Ramesh, Lane W. Martin, Long-Qing Chen, Martin V. Holt, Stephan O. Hruszkewycz and Haidan Wen, 10 July 2024, Advanced Materials.
DOI: 10.1002/adma.202405294
In addition to Wen, Holt, and Hruszkewycz, authors include Marc Zajac, Tao Zhou, Tiannan Yang, Sujit Das, Yue Cao, Burak Guzelturk, Vladimir Stoica, Mathew Cherukara, John Freeland, Venkatraman Gopalan, Ramamoorthy Ramesh, Lane Martin and Long-Qing Chen.
Funding for the research came from the DOE Office of Basic Energy Sciences.