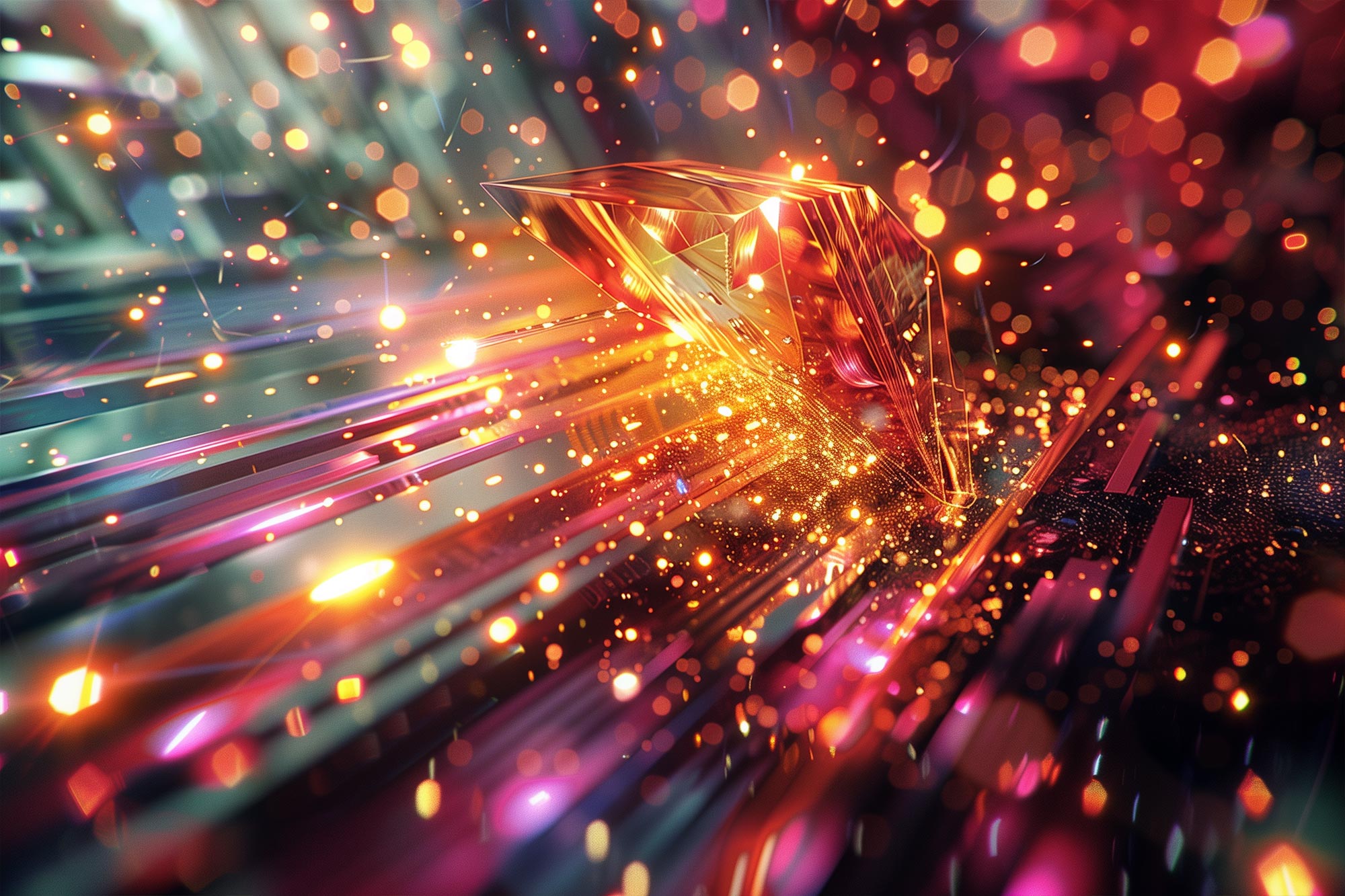
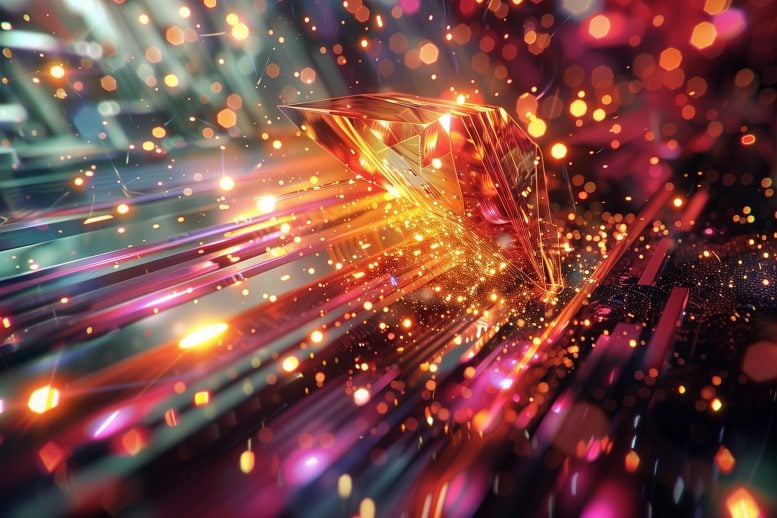
Researchers have discovered a new class of quantum critical metal, focusing on how quantum phase transitions and electronic topology affect electron behavior.
The study reveals that Kondo coupling and chiral spin liquids play a key role in this process, with potential implications for sensitive electronic devices.
Discovery of a New Class of Quantum Critical Metal
A new study led by Rice University’s Qimiao Si has revealed a novel class of quantum critical metal, illuminating the complex electron interactions in quantum materials. Published recently in Physical Review Letters, the research investigates the impact of Kondo coupling and chiral spin liquids within specific lattice structures.
“The insights gained from this discovery could lead to the development of electronic devices with extreme sensitivity, driven by the unique properties of quantum-critical systems,” said Si, the Harry C. and Olga K. Wiess Professor of Physics and Astronomy and director of Rice’s Extreme Quantum Materials Alliance.
Quantum Phase Transitions: Complex Behaviors of Electrons
At the core of this research is the concept of quantum phase transitions. Just as water changes between solid, liquid, and gas states, electrons in quantum materials can shift between different phases as their environment changes. But unlike water, these electrons follow the rules of quantum mechanics, leading to much more complex behaviors.
Quantum mechanics introduce two key effects: quantum fluctuations and electronic topology. Even at absolute zero where thermal fluctuations disappear, quantum fluctuations can still cause changes in the organization of electrons, leading to quantum phase transitions. These transitions often result in extreme physical properties known as quantum criticality.
Moreover, quantum mechanics give electrons a unique property tied to topology, a mathematical concept that when applied to electronic states can produce unusual and potentially useful behaviors.
The study was carried out by Si’s group in a long-term collaboration with Silke Paschen, study co-author and a professor of physics at the Vienna University of Technology, and her research team. Together they developed a theoretical model to explore these quantum effects.
Theoretical Model: Slow and Fast Electrons Interplay
The researchers considered two types of electrons: some moving slowly, like cars stuck in traffic, and others moving quickly in a fast lane. Although the slow-moving electrons appear stationary, their spins can point in any direction.
“Ordinarily, these spins would form an orderly pattern, but the lattice they inhabit in our model doesn’t allow for such neatness, leading to geometrical frustration,” Si said.
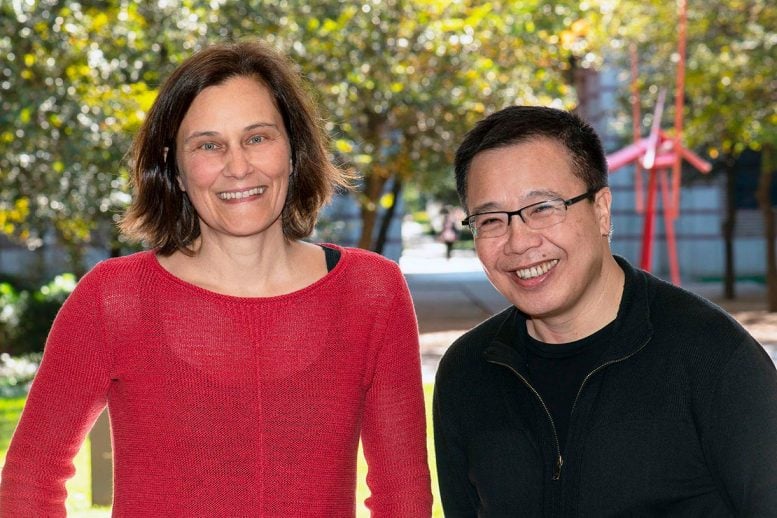
The Role of Chiral Spin Liquids and Kondo Coupling
Instead, the spins form a more fluid arrangement known as a quantum spin liquid, which is chiral and picks a direction in time. When this spin liquid couples with the fast-moving electrons, it has a topological effect.
The research team discovered that this coupling also triggers a transition into a Kondo phase, where the spins of the slow electrons lock onto the fast ones. The study reveals the complex interplay between electronic topology and quantum phase transitions.
Unusual Electrical Transport: The Hall Effect
As electrons move through these transitions, their behavior changes dramatically, particularly in how they conduct electricity.
One of the most significant findings is about the Hall effect, which describes how an electrical current bends under the influence of an external magnetic field, Paschen said.
“The Hall effect contains a component that is enabled by the electronic topology,” she said. “We show that this effect experiences a sudden jump across the quantum critical point.”
Implications for Future Technology
This discovery advances our understanding of quantum materials and opens up new possibilities for future technology. An important part of the research team’s finding is that the Hall effect responds drastically to the quantum phase transition, Si said.
“Thanks to the topology, this response happens in a minute magnetic field,” he said.
The unusual properties could lead to the development of new types of electronic devices such as sensors with extreme sensitivity that could revolutionize fields like medical diagnostics or environmental monitoring.
Reference: “Anomalous Hall Effect and Quantum Criticality in Geometrically Frustrated Heavy Fermion Metals” by Wenxin Ding, Sarah Grefe, Silke Paschen and Qimiao Si, 6 September 2024, Physical Review Letters.
DOI: 10.1103/PhysRevLett.133.106504
Co-authors of the study include Wenxin Ding of Anhui University in China, a former postdoctoral fellow in Si’s group at Rice, and Rice alumna Sarah Grefe ’17 of California State University.
The research was supported by the U.S. National Science Foundation, the Air Force Office of Scientific Research, the Robert A. Welch Foundation and a Vannevar Bush Faculty Fellowship.