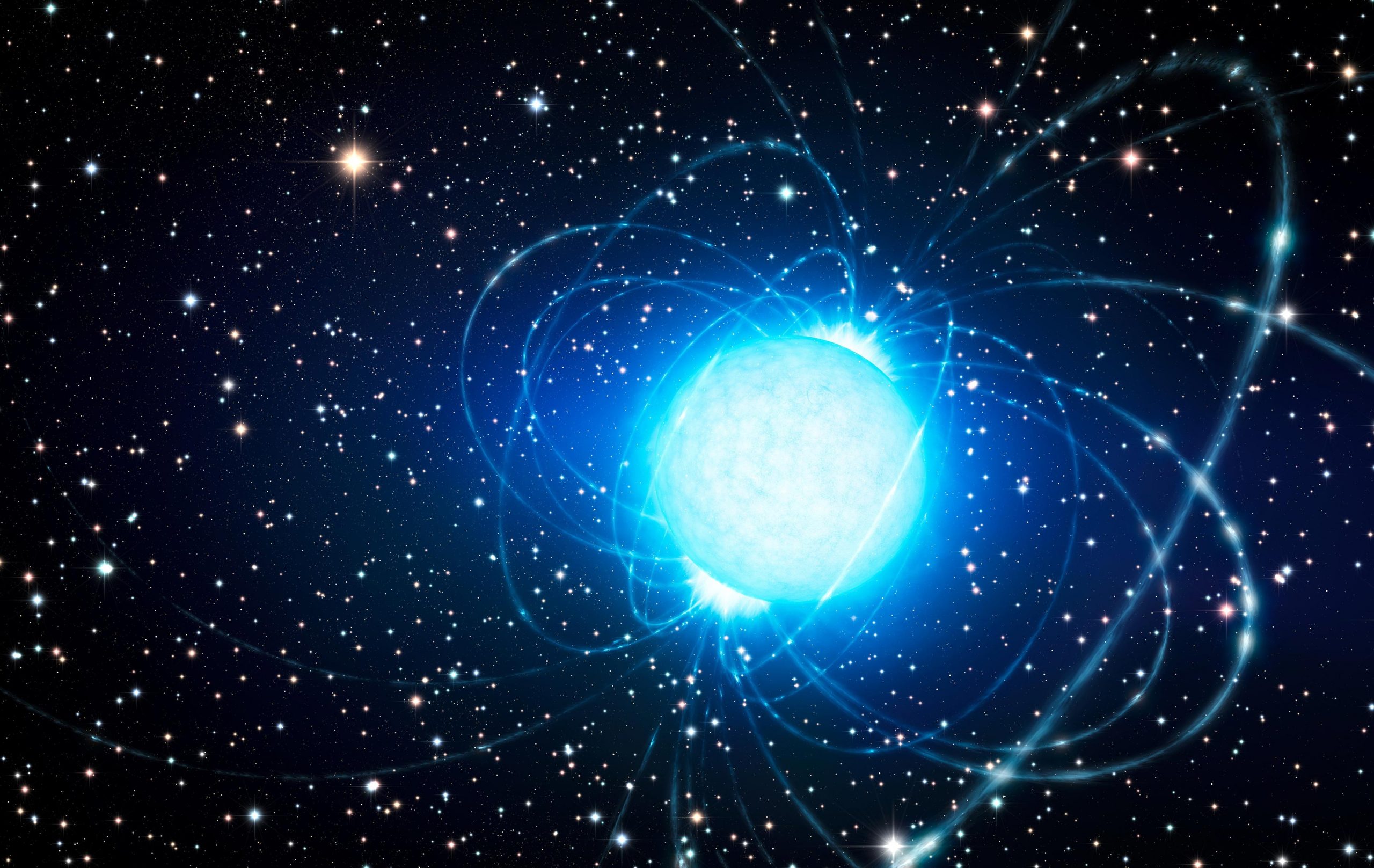
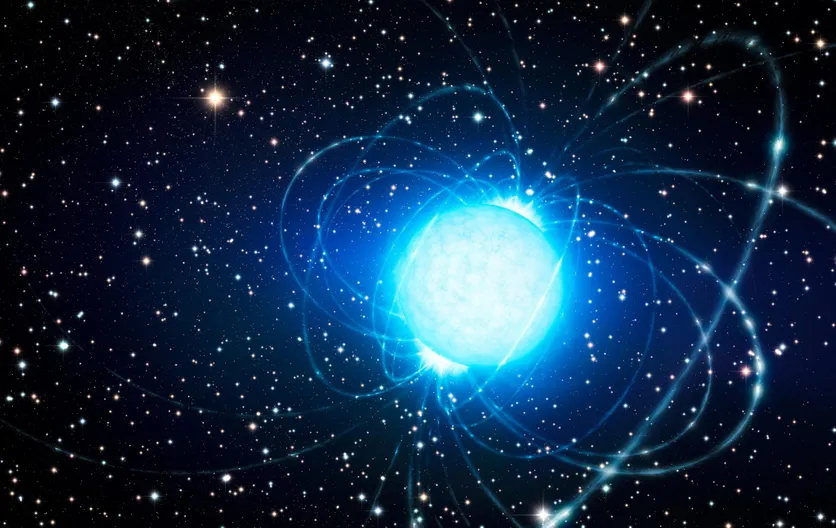
Artist’s depiction of a neutron star. Credit: ESO / L. Calçada
What Is a Neutron Star?
Neutron stars are the extremely dense remnants of supermassive stars that have exploded as supernovae.
A star’s evolution and ultimate fate depend in large part on its mass. All supermassive stars — stars with an initial mass greater than approximately eight times that of the Sun — have the capacity to eventually become neutron stars. When a supermassive star begins to die, it forms a red supergiant. After that, these stars either evolve into white dwarfs, or explode as supernovae. If what remains of the core of the star after the supernova explosion has a mass less than about three times the Sun’s mass, then it forms into a neutron star (if the remnant is more massive, it will collapse into a black hole).
Neutron stars are incredibly dense. They have a mass greater than that of the entire Sun, but are packed into a radius of only about 10 kilometers (6 miles). A single teaspoon of a neutron star would have a mass of about a trillion kilograms. Neutron stars are so named because they are composed primarily of neutrons, as most of the protons and electrons will have combined to form neutrons under the extremely dense conditions. Even though they do not actively generate heat through nuclear fusion, neutron stars are incredibly hot, with temperatures far exceeding those of regular stars.

This is a near-infrared-light image of the neutron star RX J0806.4-4123 taken with the NASA/ESA Hubble Space Telescope. Hubble detected an unusual excess of infrared radiation that might be evidence for a disc around the stellar remnant. Or it could be from a wind of charged particles streaming off the neutron star and slamming into gas in the interstellar medium the neutron star is plowing through. Credit: NASA, ESA, and B. Posselt (Pennsylvania State University)
In 1997 Hubble provided the first direct look, in visible light, at an isolated neutron star. The telescope’s results showed the star is very hot (670,000 degrees Celsius / 1,200,000 degrees Fahrenheit at the surface), and can be no larger than 28 kilometers (17 miles) across. These results proved that the object must be a neutron star, because no other known type of object can be this hot, small, and dim.
In 2017 the telescope also observed for the first time the source of gravitational waves created by the merger of two neutron stars. This merger gave rise to an event known as a kilonova — something actually predicted by theory decades ago — that results in the ejection of heavy elements such as gold and platinum into space. This event also provided the strongest evidence to date that short-duration gamma-ray bursts are caused by mergers of neutron stars. Prior to this finding, connecting kilonovae and short gamma-ray bursts to neutron star mergers had been difficult, but the multitude of detailed observations following the detection of the gravitational wave event — including those by Hubble — finally verified these connections.

Neutron Star. Credit: ESA/Hubble, NASA, ESA, and B. Posselt (Pennsylvania State University)
In May of 2020, the light from the glow of a kilonova caused by the merger of two neutron stars reached Earth. Hubble was then used to study the explosion’s aftermath and the host galaxy, and found that the near-infrared emission was 10 times brighter than predicted. These results challenged conventional theories of what happens in the aftermath of a short gamma-ray burst.